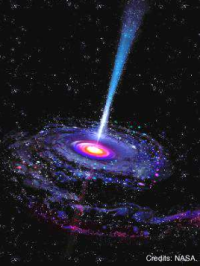
Astroparticle physics is a field of research to study
- particle physics beyond the reach of terrestrial experiments through astrophysical phenomena; and
- astrophysics beyond the reach of traditional astronomy using elementary particles such as neutrinos.
Therefore, this field has strong connection with dark matter detection experiments, high-energy astrophysics, and neutrino physics.
It has been observationally revealed that about 85% of the total matter in the Universe is made of dark matter. However, its true identity is still to be uncovered. Since dark matter cannot be explained by any particles in the Standard Model of particle physics, it is most natural to expect that it is made of undiscovered particles predicted by physics beyond the Standard Model. Popular candidates theoretically proposed but yet to be tested experimentally include neutralinos predicted by supersymmetric extension of the Standard Model, sterile neutrinos that only talk to the Standard Model sector through neutrino mixing, and axions that are introduced to solve the strong CP problem. Researchers at the Kavli IPMU explore all the possibilities, aiming at making breakthrough by discovering particle dark matter in the near future.
What is most relevant for astroparticle physics is indirect dark matter searches that aim to find a weak signal of dark matter originated from somewhere in the sky. For example, if dark matter is made of weakly interacting massive particles (the supersymmetric neutralino is one such example), they annihilate with themselves and create gamma rays, charged particles, and neutrinos, all of which can be detected with high-energy detectors.
At the Kavli IPMU, the researchers
- interpret existing data and give future prospects for new experiments, starting from particle physics modeling,
- predict signals from dark matter from the most promising regions in the sky through modeling of large-scale structure in the Universe, and
- constrain the strength of dark matter interactions by performing data analyses,
in order to approach this challenging problem.
For detecting dark matter signatures from celestial objects, one must assess and subtract other ordinary signals from high-energy astrophysical sources. Therefore, this field has strongly related to high-energy astrophysics. Conversely, new analysis techniques developed for dark matter searches are often applied to reveal new properties of high-energy astrophysical sources. Ideas from researchers at the Kavli IPMU have been adopted throughout the world to expand the horizon.
It is extremely important to use new agents besides photons such as neutrinos in order to probe astrophysical phenomena that are unknown or not well understood. For example, detecting neutrinos from supernova 1987A made it possible to probe physics of core collapse deep inside a massive star. Recent detection of gravitational waves enable precision studies of physics of black holes and neutron stars. High-energy neutrinos as well as cosmic rays are also expected to be extremely important as an entirely new probes for astrophysics. All these are important research subjects of the Kavli IPMU and researchers take comprehensive and various approaches to these highly challenging and intertwined problems.
(Last update: 2018/05/07)
Members
- Patrick De Perio
- Andrew Eberhardt
- Johannes Herms
- Katsuki Hiraide
- Junji Hisano
- Shunsaku Horiuchi
- Hirokazu Ishino
- Masahiro Kawasaki
- Takeshi Kobayashi
- Kazunori Kohri
- Alexander Kusenko
- Qiuyue Liang
- Jia Liu
- Kai Martens
- Lluis Marti Magro
- Shigeki Matsumoto
- Thomas (Tom) Melia
- Yuta Michimura
- Shinji Mukohyama
- Hitoshi Murayama
- Shoei Nakayama
- Yasunori Nomura
- Sebastien Peirani
- Katsuhiko Sato
- Hiroyuki Sekiya
- Yevgeny Stadnik
- Masahiro Takada
- Tadayuki Takahashi
- Volodymyr Takhistov
- Leander Thiele
- Mark Vagins
- Masaki Yamashita
- Tsutomu Yanagida
- Vicharit Yingcharoenrat
- Naoki Yoshida