10 May, 2023
RIKEN
Japan Atomic Energy Agency
Tokyo Metropolitan University, Rikkyo University
Kastler Brossel Laboratory
Kavli Institute for the Physics and Mathematics of the Universe (Kavli IPMU), The University of Tokyo
KEK
J-PARC
Chubu University
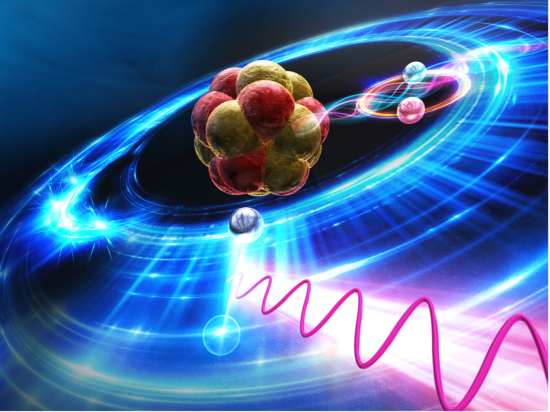
An international collaboration of researchers, including the Kavli Institute for the Physics and Mathematics of the Universe (Kavli IPMU), has succeeded in a proof-of-principle experiment to verify strong-field quantum electrodynamics[4] with exotic atoms[5], by performing high-precision measurements of the energy spectrum of muonic characteristic X-rays[1] emitted from muonic atoms[2] using a state-of-the-art X-ray detector, reports a new study in Physical Review Letters.
The group's results are a significant step toward verifying fundamental physical laws under strong electric fields, which humankind has not yet been able to create artificially. The highly efficient and accurate X-ray energy determination method using state-of-the-art quantum technology demonstrated in this research is expected to be applied to various research fields, such as non-destructive elemental analysis methods using muonic atoms[6].
It has always been a dream of scientists to discover physical laws. They have been found or proposed to explain observed phenomena that cannot be understood by existing theories. In many cases, the discovery of new physics requires the development of new experimental techniques and improved accuracy of measurements. The most precisely tested theory of physical laws is Quantum ElectroDynamics (QED), which describes the microscopic interactions between charged particles and light. Scientists are constantly pushing the limits of how far QED accurately describes our physical reality.
In this paper, the collaboration, including Dr. Takuma Okumura (Postdoctoral Researcher, RIKEN at the time of the research, now Assistant Professor at Tokyo Metropolitan University), Professor Toshiyuki Azuma (Chief Scientist, RIKEN), Dr. Tadashi Hashimoto (Assistant Principal Researcher) of Japan Atomic Energy Agency (JAEA), Visiting Researcher Hideyuki Tatsuno of Tokyo Metropolitan University, Associate Professor Shinya Yamada of Rikkyo University, Professor Paul Indelicato of the Kastler-Brossel Laboratory, Professor Tadayuki Takahashi of the Kavli Institute for the Physics and Mathematics of the Universe, the University of Tokyo, Professor Koichiro Shimomura of Institute for Materials Structure Science, KEK, Professor Shinji Okada of Chubu University, injected a low-velocity negative muon beam from the J-PARC[7] facility into neon gas, and the energy of characteristic X-rays emitted from the resulting muonic neon (Ne) atoms was precisely measured using a superconducting Transition-Edge Sensor (TES)[3] detector. By taking full advantage of the excellent energy resolution of the TES detector, the energy of the muonic characteristic X-rays was determined with an absolute uncertainty of less than 1/10,000, and contributions from vacuum polarization[8] in strong-field quantum electrodynamics were successfully verified with a high precision of 5.8 %.
The TES detector was originally developed for space X-ray observation. Takahashi’s current project at Kavli IPMU has been to carry out unprecedented cross-disciplinary research using this detector. His team includes Kavli IPMU Project Assistant Professor Shin'ichiro Takeda, Project Researcher Miho Katsuragawa, and at-the-time graduate student Kairi Mine, who took part in the muon experiments.
The collaboration’s demonstration of the experimental technique using muonic atoms is expected to lead to a great leap forward in the study of QED verification under strong electric fields.
Details of the study were published online (27 April 2023 Japan time) prior to publication in the scientific journal Physical Review Letters (27 April 2023 Japan time).
Background
The effects of QED are more pronounced in environments with strong electric fields, but theoretical calculations become more difficult in this case. Therefore, a strong electric field environment is very important for QED verification. For many years, experiments using highly charged ions (HCIs), which are atoms stripped of multiple electrons, have been conducted as an approach to realize a strong electric field environment. The electric field felt by the bound electrons in HCIs becomes stronger as the atomic number becomes larger, and the shielding effect[10] is suppressed by the stripping of many electrons. HCI research using large accelerators is still vigorously pursued. However, even for HCIs with large atomic numbers, the effect of the finite size of the nucleus cannot be ignored. It has been pointed out that this effect is not precisely known, and thus the accuracy of QED verification, which compares experimental results with theory, is greatly compromised.
Research Methodology and Results
To verify QED under strong electric fields in a different way than with HCIs, international research groups have focused on "exotic atoms," in which a negatively charged particle is bound to the nucleus instead of the electron. Among the variety of exotic atoms, muonic atoms are composed of negative muons (elementary particles about 200 times heavier than electrons) and nuclei. Negative muons can nowadays be extracted as beams from large accelerators. Muonic atoms are characterized by the extremely close proximity of the negative muon to the nucleus, with the orbital radius of a bound muon being approximately 1/200th that of a bound electron. As a result, the electric field felt by the muon is about 40,000 times stronger than the electric field felt by a bound electron of the same quantum level in an HCI, resulting in a huge QED effect. In addition, by using negative muons, which occupy high angular momentum quantum levels with small overlap with the nucleus, it is possible to conduct experiments in which the effect of the finite size of the nucleus is largely suppressed. By precisely measuring the energy of muonic characteristic X-rays emitted when muonic atoms are deexcited from a specific level to lower levels, QED can be verified under a strong electric field (Figure 2).
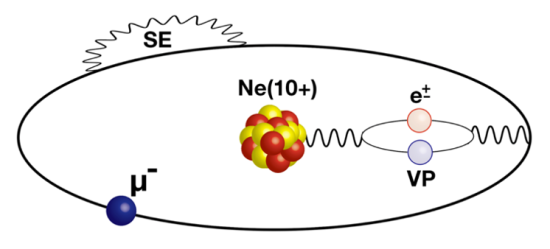
Thus, muonic atoms are a promising experimental target for strong-field QED verification. However, there are several problems to overcome. The largest is that a number of muonic atoms must be prepared in an isolated environment. The presence of atoms or molecules in the vicinity of the muonic atoms may cause rapid electron transfer and changes the energy of the muonic characteristic X-rays. The solution is to use dilute gas targets with a small number density (low pressure), but the number of produced muonic atoms and the resulting intensity of muonic characteristic X-rays are reduced. The international research group conducted experiments at the Japan Proton Accelerator Research Complex (J-PARC) in Tokai-mura, Ibaraki, where the world's most intense low-velocity muon beam is available. In order to determine the energy with sufficient accuracy even with low-intensity muonic characteristic X-rays, the experiment was conducted with a superconducting transition edge sensor (TES) microcalorimeter, which is a highly efficient and high-resolution X-ray detector.
Using rare gas neon (10Ne) atoms as the target, they have achieved an energy resolution that is one order of magnitude higher than that of conventional semiconductor detectors (FWHM[11]: 5.2 eV) under dilute conditions of 0.1 atm and successfully measured the muonic characteristic X-rays (Figure 3). The peaks shown are mainly due to the overlap of muonic characteristic X-rays from six different transitions, and the energy of the muonic characteristic X-rays was determined to a high accuracy of 0.002% by analyzing contributions from each of them.
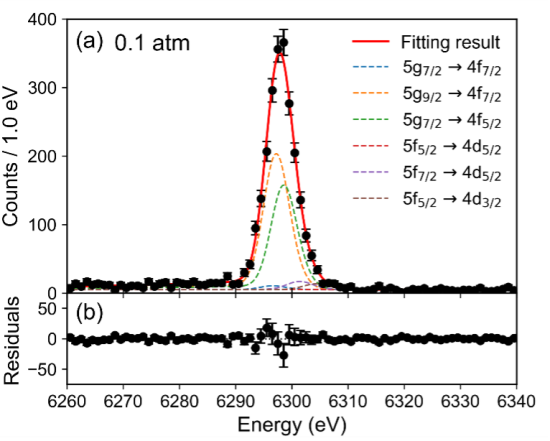
They repeated the measurements while changing the pressure of the neon gas target (Figure 4) and confirmed that the energy of the muonic X-rays is constant within experimental error regardless of the pressure of the neon gas target. Thus, it can be concluded that the muonic neon atoms were in an isolated environment. They compared the latest theoretical calculations with the experimental results and confirmed that they agreed within the experimental error. We succeeded in verifying the effect of vacuum polarization under a strong electric field with an extremely high accuracy of 5.8%. This is comparable to the accuracy of strong-field QED using the multiply charged uranium ion U91+, which is the most accurate observation to date.
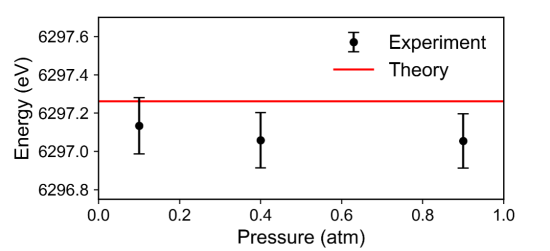
The energy of muonic characteristic X-rays is plotted against the pressure of the neon gas target. No pressure-dependent energy variation was observed within experimental error, indicating that the muon-neon atoms were in an isolated environment. The precise muonic characteristic X-ray energy measurements are in excellent agreement with the results from the latest theoretical calculations.
Paper details
Journal: Physical Review Letters
Paper title: Proof-of-Principle Experiment for Testing Strong-Field Quantum Electrodynamics with Exotic Atoms: High Precision X-ray Spectroscopy of Muonic Neon
Authors: Takuma Okumura, Toshiyuki Azuma, Douglas A. Bennett, I-Huan Chiu, William B. Doriese, Malcolm S. Durkin, Joseph W. Fowler, Johnathon D. Gard, Tadashi Hashimoto, Ryota Hayakawa, Gene C. Hilton, Yuto Ichinohe, Paul Indelicato, Tadaaki Isobe, Sohtaro Kanda, Miho Katsuragawa, Naritoshi Kawamura, Yasushi Kino, Kairi Mine, Yasuhiro Miyake, Kelsey M. Morgan, Kazuhiko Ninomiya, Hirofumi Noda, Galen C. O'Neil, Shinji Okada, Kenichi Okutsu, Nancy Paul, Carl D. Reintsema, Dan R. Schmidt, Koichiro Shimomura, Patrick Strasser, Hirotaka Suda, Daniel S. Swetz, Tadayuki Takahashi, Shinichiro Takeda, Satoshi Takeshita, Motonobu Tampo, Hideyuki Tatsuno, Yasuhiro Ueno, Joel N. Ullom, Shin Watanabe, and Shinya Yamada
DOI: 10.1103/PhysRevLett.130.173001 (published 27 April, 2023)
Paper abstract (Physical Review Letters)
Media contact
Motoko Kakubayashi
Press officer,
Kavli Institute for the Physics and Mathematics of the Universe, The University of Tokyo
Tel: 080-4056-2767
Email: press_at_ipmu.jp
* Please change _at_ to @
Supplementary Explanation
[1] Muonic characteristic X-ray
When a negative muon bound to a nucleus deexcites from a higher energy orbital to a lower energy orbital, the excess energy is emitted as X-rays. These X-rays have specific energies depending on the atom and are called muonic characteristic X-rays.
[2] Muonic atom, negative muon
Muons are one of the elementary particles and are the second generation of charged leptons in the Standard Model. There are positively or negatively charged muons, all with 1/2 spin and an average lifetime of 2.2 microseconds. Negative muons decay into electrons, muon neutrinos, and antielectron neutrinos through weak interactions. Negative muons are about 200 times heavier than electrons. Since negative muons have the same negative charge as electrons, they behave as "heavy electrons" bound to a positively charged nucleus, like electrons. An atom composed of a negative muon and a nucleus is called a muonic atom. A muonic atom eventually decays due to the lifetime of the negative muon or due to the capture of the negative muon by the nucleus.
[3] Superconducting Transition Edge Microcalorimeter (TES)
A microcalorimeter is a detector that determines the X-ray energy by the temperature rise when a material absorbs X-rays. The Superconducting Transition Edge Microcalorimeter measures the temperature change due to X-ray absorption using the steep change in electrical resistance near the superconductivity-normal conduction phase transition. TES stands for Transition-Edge Sensor.
[4] Quantum electrodynamics (QED)
QED is a field theory that regards electromagnetic interactions between charged particles as being caused by the exchange of photons and has succeeded in explaining a variety of phenomena that cannot be understood by relativistic quantum mechanics.
[5] Exotic atoms
Atoms formed by replacing the nucleus and electrons that make up an atom with other charged particles (positrons, muons, antiprotons, pions, etc.) are called exotic atoms. The combination of charge and mass of the particles that make up an exotic atom is very different from that of electrons and nuclei, so exotic atoms behave in a unique way that ordinary atoms do not.
[6] Non-destructive elemental analysis method using muon atoms
As a non-destructive elemental analysis method for unknown samples, the measurement of electronic characteristic X-rays emitted when a sample is irradiated with high-energy X-rays or electron beams has been widely used. However, the electronic characteristic X-rays emitted from light elements such as carbon and hydrogen have low energy, making it difficult to perform elemental analysis with sufficient accuracy. Recently, a non-destructive elemental analysis method using muonic characteristic X-rays, which are emitted when a sample material is irradiated with a negative muon beam, has been established. Since muonic characteristic X-rays generally have higher energy than electronic characteristic X-rays, this method has an extremely high sensitivity to light elements. The muon-based elemental analysis method has been used for the analysis of samples brought back from the asteroid "Ryugu," and its usefulness has been demonstrated.
[7] J-PARC (Japan Proton Accelerator Research Complex)
J-PARC is an accelerator facility located in Tokai-mura, Ibaraki, where advanced research in various fields, such as particle and atomic physics, materials, and life sciences, is conducted using the world's most intense proton beams. The Muon Science Experimental Facility MUSE is in the Materials and Life Science Experimental Facility (MLF) at J-PARC.
[8] Vacuum polarization
Quantum electrodynamic effects on muon atomic energy can be classified into two main types: vacuum polarization and self-energy. According to quantum electrodynamics, photons, which are responsible for electromagnetic interactions, are constantly repeating the creation and annihilation reactions of virtual electron-positron pairs. The energy change associated with the creation and annihilation of these virtual electron-positron pairs is called vacuum polarization. Bound muons in muonic atoms are constantly absorbing and emitting virtual photons, and the energy change due to this process is called self-energy. It is known that the effect of vacuum polarization is particularly significant in quantum electrodynamic effects on muonic atoms, and in the case of muonic neon atoms, the effect of vacuum polarization is more than 1,000 times larger than the self-energy.
[9] Transition energy
According to quantum mechanics, muons and electrons bound to nuclei occupy discrete orbits, with their energy also being discretized (quantized). The extra energy produced when a particle transitions from a higher energy orbital to a lower energy orbital is called the transition energy. In many cases, the transition energy is emitted as electromagnetic waves (light or X-rays).
[10] Shielding effect
In an atom with multiple electrons, the effect is that the Coulomb force between the electrons and the nucleus appears to be reduced due to the charge of the remaining electrons.
[11] Half-width
This indicates the width at half the value of an observation. The smaller this value is, the higher the resolution of the observation and the more precise the peak position can be measured.
[12] Schwinger limit
According to quantum electrodynamics, in very strong electric fields exceeding an electric field strength of 1.32 x 1018 V/m, the electromagnetic field exhibits nonlinear effects. This limiting electric field is called the Schwinger limit after the physicist Julian Schwinger.
Related links
Toward new frontiers : Encounter and synergy of state-of-the-art astronomical detectors and exotic quantum beams
International Collaboration Group
This research was based on experimental results by an international research group consisting of RIKEN, NIST (National Institute of Standards and Technology), Tokyo Metropolitan University, Rikkyo University, Kastler Brossel Laboratory (France), KEK (High Energy Accelerator Research Organization), Tohoku University, JAXA (Japan Aerospace Exploration Agency), and Chubu University. The experiment was conducted at the Materials and Life Science Experimental Facility (MLF) of the Japan Proton Accelerator Research Complex (J-PARC), which is jointly operated by the Japan Atomic Energy Agency (JAEA) and the High Energy Accelerator Research Organization (KEK). RIKEN and Chubu University played a leading role in the overall research, while NIST played a key role in the development of the detector. The High Energy Accelerator Research Organization (KEK) Institute for Materials Structure Science developed and operated the low-velocity negative muon beam and played an important role in the installation of experimental equipment, while the Kavli Institute for the Physics and Mathematics of the University of Tokyo, Osaka University, JAEA, Tokyo Metropolitan University, Rikkyo University, Kastler Brossel Laboratory in France, Tohoku University, and JAXA joined the development of experimental equipment and measurement systems, and the acquisition and analysis of experimental data.
Research Support
This research was funded by the Japan Society for the Promotion of Science (JSPS) Grant-in-Aid for Scientific Research on Innovative Areas (Research Area Proposal Type), "Toward news frontiers: Encounter and synergy of state-of-the-art astronomical detectors and exotic quantum beams (PI: Tadayuki Takahashi)," "Precise verification of atomic and molecular physics by negative muon beam and its application to astrophysical observations (PI: Toshiyuki Azuma)," Grant-in-Aid for Scientific Research (A), "Clarification of Thorium-229 nuclear isomer structure: new development of high precision clock science (PI: Tadaaki Isobe)," Grant-in-Aid for Scientific Research (A), "Innovation of atomic and molecular dynamics research using superconductive molecular detectors (PI: Shinji Okada)," Grant-in-Aid for Challenging Research (Pioneering), "Development of cryogenic Compton camera for high precision x-ray polarization spectroscopy (PI: Shinya Yamada), "Grant-in-Aid for Young Scientists, "Chemical reactions of negative ions in space environments probed by advanced neutral molecular detectors (PI: Takuma Okumura)," and Grant-in-Aid for Transformative Research Areas (A) (Publicly Offered Research), "Development of next-generation neutral molecular detection system for astrochemical reactions (PI: Takuma Okumura), and the RIKEN Pioneering Projects funded by RIKEN.