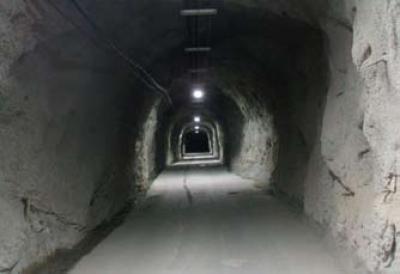
What are the building blocks of nature? Most people have heard of electrons, which are indeed (as far as we can tell) fundamental particles, as well as protons and neutrons, which are themselves composite objects composed of much smaller fundamental particles called quarks. But there are much more unusual fundamental particles, too, and perhaps the most mysterious of these are the neutrinos.
The Standard Model of particle physics contains three generations of fundamental particles. In each of these generations, or families, there are two quarks and two much less massive particles called leptons. In the first family one such lepton is the electron, which carries an electric charge, and the other first-generation lepton is called the electron neutrino, which is electrically neutral. The second generation contains two more types of quarks, a charged lepton called the muon, and the muon neutrino, while the third family contains a final pair of quarks, a charged lepton called the tau, and a tau neutrino.
The three types of neutrinos, the electron neutrino, the muon neutrino, and the tau neutrino, are exceedingly challenging to study, because they hardly interact with matter at all. That means neutrino detectors need to be very big, very sensitive, or both. At IPMU we have teams of researchers working on some of the best and most famous neutrino detectors and experiments in the world.
The Super-Kamiokande [Super-K] detector is a 50,000 ton tank of water buried deep under the Japanese Alps. By studying neutrinos generated by cosmic ray interactions in the Earth’s atmosphere, in 1998 Super-K made the stunning discovery that different types of neutrinos can spontaneously transform from one type to another, a process known as neutrino oscillation. This also implied that at least two of the three neutrinos have a small, but non-zero mass, something not predicted by the Standard Model. This was the first time since its inception that the Standard Model needed to be revised based on solid experimental data. In 2001 Super-K made a crucial contribution to the solution of the solar neutrino problem by indicating that solar neutrinos produced by the Boron-8 reaction in the Sun could change their flavor while in flight, and uniquely selected the large mixing angle solution to the problem. The 2015 Nobel Prize in Physics was awarded for these discoveries. Kavli IPMU members are now leading the effort on GADZOOKS!, also known as SK-Gd, an initiative to enrich the ultrapure water inside Super-Kamiokande with the element gadolinium. This will greatly reduce backgrounds and, among many other physics benefits, should allow the first-ever detection of a constant stream of neutrinos from distant supernovas. In the summer and fall of 2018 the detector was refurbished in preparation for the addition of gadolinium, which could occur as soon as the second half of FY2019.
The KamLAND neutrino detector is located in the same ancient zinc mine as Super-Kamiokande, but instead of water it is filled with 1,000 tons of liquid scintillator. This makes it very sensitive, especially to low energy neutrinos from nuclear reactors and those generated by radioactive decays within the Earth itself. In 2002 KamLAND was the first experiment to observe disappearance of reactor neutrinos, which matched other experiments’ solar neutrino data in spectacular fashion. After lowering the energy threshold at which their data could be analyzed, in 2005 KamLAND was the first experiment to detect geoneutrinos, ushering in an entirely new way to study the Earth’s interior. Also in 2005, KamLAND saw evidence of spectral distortions in the reactor neutrino signal; clear proof of neutrino oscillations. Since the year 2011, the KamLAND detector was modified into a huge neutrinoless double beta decay experiment via the addition of Xenon-136 to the detector volume. In May 2018, a new phase of the experiment called KamLAND-Zen 800 began. The KamLAND-Zen collaboration completed construction of the detector containing 800kg of Xenon-136 (more than 2 times larger than the previous phase) in August 2018.
The T2K (Tokai-to-Kamioka) experiment uses beam of neutrinos produced with a particle accelerator and detected in the Super-Kamiokande detector to study the oscillations of neutrinos. A beam consisting of one type of neutrinos, called muon neutrinos, is produced at the J-PARC accelerator located in Tokai-mura, Ibaraki-ken. A very small fraction of the produced neutrinos are detected in the Super-Kamiokande detector, which is located 295 km away from the neutrino source. In 2013, the T2K experiment showed that some of the muon neutrinos change their type to electron neutrinos for the first time. T2K is now studying the same process for antineutrinos, the antimatter partners of neutrinos. If T2K finds that neutrinos and antineutrinos make this transition at a different rate, it may help explain why the universe is composed of matter and not equal amounts of matter and antimatter. The Kavli IPMU members are leading the efforts to analyze the T2K data and search for differences (CP asymmetries) in the oscillations of neutrinos and antineutrinos. Recent results show a hint of asymmetry between neutrinos and antineutrinos that may be confirmed with additional data.
Neutrinos are deeply involved in the origin and evolution of the Universe in their nature (CP violation of their oscillations), as well as the various processes which produce them within the Earth, upon the Earth, above the Earth, within the Sun, and inside exploding stars. The Kavli IPMU researchers are probing the most inaccessible and farthest reaches of the Universe itself, using these tiniest of particles, whose understanding has been recently advanced but still they are mysterious.
(Last update: 2019/08/20)
Links
Members
- Shin'ichiro Ando
- Patrick De Perio
- Sanshiro Enomoto
- Shao-Feng Ge
- Mark Hartz
- Yoshinari Hayato
- Johannes Herms
- Katsuki Hiraide
- Junji Hisano
- Shunsaku Horiuchi
- Hirokazu Ishino
- Takaaki Kajita
- Ryuichiro Kitano
- Kazunori Kohri
- Alexander Kusenko
- Jia Liu
- Kai Martens
- Lluis Marti Magro
- Shigetaka Moriyama
- Shinji Mukohyama
- Hitoshi Murayama
- Masayuki Nakahata
- Kenzo Nakamura
- Tsuyoshi Nakaya
- Shoei Nakayama
- Serguey Petcov
- Hiroyuki Sekiya
- Masahiro Takada
- Yasuhiro Takemoto
- Volodymyr Takhistov
- Ka Ming Tsui
- Mark Vagins
- Christopher Walter
- Masaki Yamashita
- Tsutomu Yanagida
- Masashi Yokoyama
- Tianyu Zhu